The Quantum Reform of the Modern Metric System:
Mass becomes quantum, and electrical measurements become honest
Professor William Phillips, University of Maryland College Park
Tuesday, October 22, 2024
1101 A. James Clark Hall, University of Maryland College Park
Abstract: The modern metric system, officially known as the International System of Units (SI), has recently undergone its most revolutionary change since the inception of the metric system at the time of the French revolution. The kilogram, ampere, kelvin, and mole all have new definitions, based on fixing the values of fundamental constants of nature. Famously, the reform eliminates the artifact International Prototype Kilogram as the foundation of the unit of mass. This lecture will explain why this was necessary and how it was accomplished. Less famously, the century-old practice of having two separate unit systems for electrical metrology —“legal” and “scientific”— has disappeared with the reformed SI.
Biography:
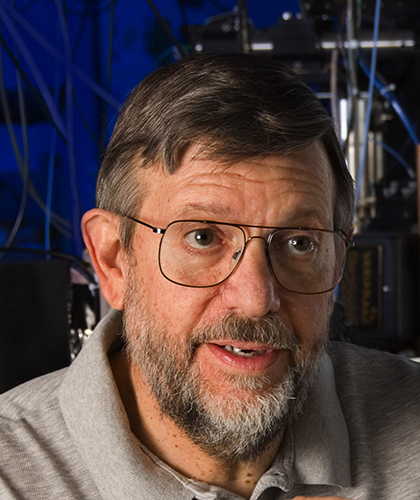
William Phillips is a Distinguished University and College Park Professor of physics. In 1997 he was a co-recipient of the Nobel Prize of Physics "for development of methods to cool and trap atoms with laser light."
Professor Phillips received his B.S. in 1970 from Juniata College and his Ph.D. from the Massachusetts Institute of Technology. He is a Fellow of the American Physical Society, a Fellow and Honorary Member of the Optical Society of America and a Member of the National Academy of Sciences.
His research interests include: Laser cooling and trapping of neutral atoms; quantum information with single-atom qubits; atomic-gas Bose-Einstein condensates; atoms in optical lattices; atomic physics analogs of condensed matter systems; coherent deBroglie-wave atom optics; and collisions of ultracold atoms.
Learn how NASA's James Webb Space Telescope was built and what the implications of its data are for discoveries about our universe
John Mather, College Park Professor of Physics and NASA Senior Scientist
NASA, Goddard Space Flight Center, Greenbelt, Maryland
Tuesday, October 17, 2023
1101 A. James Clark Hall, University of Maryland
Abstract
The James Webb Space Telescope was launched on December 25, 2021, with commissioning complete in early July 2022. With its 6.5-meter golden eye, cameras and spectrometers, the Webb Telescope is already producing magnificent images of galaxies, active galactic nuclei, star-forming regions, and planets.
Scientists are beginning to observe the growth of galaxies, the formation of stars and planetary systems, individual exoplanets through coronagraphy and transit spectroscopy, and all objects in the Solar System from Mars and beyond. In this year's Paint Branch Lecture, John Mather will explain how he and his team built the Webb Telescope and the discoveries they hope to make with its data. The Webb Telescope is a joint project of NASA with the European and Canadian space agencies.
Biography
Dr. John C. Mather is a Senior Astrophysicist in the Observational Cosmology Laboratory at NASA's Goddard Space Flight Center. His research centers on infrared astronomy and cosmology. As an NRC postdoctoral fellow at the Goddard Institute for Space Studies (New York City), he led the proposal efforts for the Cosmic Background Explorer (74-76), and came to GSFC to be the Study Scientist (76-88), Project Scientist (88-98), and also the Principal Investigator for the Far IR Absolute Spectrophotometer (FIRAS) on COBE. He showed that the cosmic microwave background radiation has a blackbody spectrum within 50 ppm. As Senior Project Scientist (95-present) for the James Webb Space Telescope, he leads the science team, and represents scientific interests within the project management. He has served on advisory and working groups for the National Academy of Sciences, NASA, and the NSF (for the ALMA, the Atacama Large Millimeter Array, and for the CARA, the Center for Astrophysical Research in the Antarctic). He has received many awards including the Nobel Prize in Physics, 2006, for his precise measurements of the cosmic microwave background radiation using the COBE satellite.
How Quantum Mechanics Helps Identify Mechanisms and Discover Materials to Combat Climate Change
Prof. Emily A. Carter, Princeton University and Princeton Plasma Physics Laboratory
Tuesday, October 25, 2022
Abstract
Each year that passes now brings more evidence that carbon dioxide and methane emissions are bringing our world closer to a tipping point beyond which it is unclear how humans and other living creatures will survive. I have been driven by this concern for the last 15 years. I decided a decade and a half ago to reorient all my research to work on sustainable energy solutions. I am a physical chemist by training, with expertise in quantum mechanics methods development and applications primarily related to materials science and chemistry. While I still work on sustainable energy, it is now evident that such solutions are not enough. We must stop emitting additional carbon into the atmosphere; we must go not just to zero but to negative emissions,1 in order to mitigate against worsening climate change caused by nearly a century and a half of fossil fuel extraction and burning. Thus, in addition to materials discovery for sustainable, carbon-free electricity (e.g., solar cells, fusion, solid oxide fuel cells), I work on catalyst discovery for renewable fuels, via (electro/solar thermo)-chemical water splitting and (photo/electro/solar thermo)-chemical carbon dioxide reduction. If we can produce liquid fuels from carbon dioxide and water – effectively running combustion backwards – via innovative catalysts that take in sunlight or carbon-free excess electricity, we will recycle carbon rather than extract and add more of it to the atmosphere and oceans. However, recycling carbon dioxide and methane is still not enough. We must convert and store carbon dioxide and methane permanently. Rather than focus on subsurface sequestration of carbon dioxide, let’s find more productive – and less risky – uses for CO2. In this talk, I will describe research related to carbon cycling back to fuels and useful chemicals, along with a vision for getting to negative emissions enabled by electrocatalysis. These approaches, along with available and emerging strategies in agriculture, forestry, and construction materials,2 may let our grandchildren and their descendants still enjoy the world we have been lucky enough to inhabit up to now. I hope that my talk will inspire others to join the effort.
1 See this report I coauthored five years ago: https://www.energy.gov/seab/downloads/final-report-task-force-co2-utilization
2 C. Hepburn, E. Adlen, J. Beddington, E. A. Carter, S. Fuss, N. MacDowell, J. C. Minx, P. Smith, and C. Williams. "The technological and economic prospects for CO2 utilization and removal," Nature 575, 87 (2019). doi: 10.1038/s41586-019-1681-6.
Order, Disorder and the Entropic Bond: The Truth about Entropy
Dr. Sharon Glotzer of the University of Michigan
Tuesday, October 12, 2021
Abstract
Entropy is typically associated with disorder; yet, the counterintuitive notion that particles with no interactions other than excluded volume might self-assemble from a fluid phase into an ordered crystal has been known since the mid-20th century. First predicted for rods, and then spheres, the thermodynamic ordering of hard particle shapes by nothing more than crowding is now well established. In recent years, surprising discoveries of entropically ordered colloidal crystals of extraordinary structural complexity have been predicted by computer simulation and observed in the laboratory. Colloidal quasicrystals, clathrate structures, and structures with large and complex unit cells typically associated with metal alloys can all self-assemble from disordered phases of identical nanoparticles due solely to entropy maximization. In this talk, we show how entropy alone can produce order and complexity beyond that previously imagined. We introduce the notion of the entropic bond, and show how methods used by the quantum community to predict atomic crystal structures can be used to predict entropic colloidal crystals
Biography
Sharon C. Glotzer is the Anthony C. Lembke Department Chair of Chemical Engineering, John Werner Cahn Distinguished University Professor of Engineering and the Stuart W. Churchill Collegiate Professor of Chemical Engineering, and Professor of Materials Science and Engineering, Physics, Applied Physics, and Macromolecular Science and Engineering at the University of Michigan in Ann Arbor. She is a member of the National Academy of Sciences, the National Academy of Engineering, the American Academy of Arts and Sciences, and a fellow of the American Association for the Advancement of Science, the American Physical Society, the American Institute of Chemical Engineers, the Materials Research Society, and the Royal Society of Chemistry. She received her B.S. degree from the University of California, Los Angeles, and her Ph.D. degree from Boston University, both in physics. Prior to joining the University of Michigan in 2001, she worked for eight years at the National Institute of Standards and Technology where she was co-founder and Director of the NIST Center for Theoretical and Computational Materials Science.
Professor Glotzer’s research on computational assembly science and engineering aims toward predictive materials design of colloidal and soft matter, and is sponsored by the NSF, DOE, DOD and Simons Foundation. Among her notable findings, Glotzer’s introduction of the notion of “patchy particles,” a conceptual approach to nanoparticle design, has informed wide-ranging investigations of self-assembly. She showed that entropy alone can assemble shapes into many structures, which has implications for materials science, thermodynamics, mathematics, and nanotechnology. Her group’s “shape space diagram” shows how matter self-organizes based on the shapes of the constituent elements, making it possible to predict what kind of material—glass, crystal, liquid crystal, plastic crystal, or quasicrystal—will emerge.
Glotzer runs a large computational research group of 30 students, postdocs, and research staff, and has published over 270 refereed papers and presented over 300 plenary, keynote and invited talks around the world. She has provided leadership and input on roadmapping for federal granting agencies on many research topics, and has been a leading advocate for simulation-based materials research, including nanotechnology and high performance computing, serving on boards and advisory committees of the National Science Foundation, the U.S. Department of Energy, and the National Academies. She is currently a member of the National Academies Board on Chemical Sciences and Technology. She is a Simons Investigator, a former National Security Science and Engineering Faculty Fellow, and the recipient of numerous other awards and honors, including the 2019 Aneesur Rahman Prize for Computational Physics from the American Physical Society, the 2018 Nanoscale Science and Engineering Forum and the 2016 Alpha Chi Sigma Awards both from the American Institute of Chemical Engineers, and the 2017 Materials Communications Lecture Award and 2014 MRS Medal from the Materials Research Society.
Due to Covid, there was no Paint Branch Lecture in 2020.
From Nonlinear Optics to High-Intensity Laser Physics
Donna Strickland
Thursday, September 19, 2019
Abstract
The laser increased the intensity of light that can be generated by orders of magnitude and thus brought about nonlinear optical interactions with matter. Chirped pulse amplification, also known as CPA, changed the intensity level by a few more orders of magnitude and helped usher in a new type of laser-matter interaction that is referred to as high-intensity laser physics. In this talk, I will discuss the differences between nonlinear optics and high-intensity laser physics. The development of CPA and why short, intense laser pulses can cut transparent material will also be included. I will also discuss future applications.
Biography
Dr. Donna Strickland is on
e of the recipients of the Nobel Prize in Physics 2018 for co-inventing Chirped Pulse Amplification with Dr. Gérard Mourou, her PhD supervisor at the time of the discovery. She earned her PhD in optics from the University of Rochester and her B. Eng. from McMaster University. Dr. Strickland was a research associate at the National Research Council Canada, a physicist at Lawrence Livermore National Laboratory and a member of technical staff at Princeton University. In 1997, she joined the University of Waterloo, where her ultrafast laser group develops high-intensity laser systems for nonlinear optics investigations. She is a recipient of a Sloan Research Fellowship, a Premier’s Research Excellence Award and a Cottrell Scholar Award. She served as the president of the Optical Society (OSA) in 2013 and is an OSA Fellow and an SPIE Fellow.
Capturing Light on the Nanoscale
John B. Pendry
Tuesday, October 23, 2018
Abstract
Convention has it that light cannot resolve objects much smaller than the wavelength, a ‘rule’ that was shown to be invalid some years ago [1]. Here we look inside the wavelength and study the properties of plasmonic structures with dimensions of just a few nanometres: a tenth or even a hundredth of the wavelength of visible light, where the ray picture of light fails utterly. We show how the new concept of transformation optics that manipulates electric and magnetic field lines rather than rays can provide an equally intuitive understanding of sub wavelength phenomena and at the same time be an exact description at the level of Maxwell’s equations. The new technology brings light into contact with the nanoworld.
[1] Negative Refraction Makes a Perfect Lens, Phys. Rev. Lett. 85, 3966-9 (2000).
Biography
John Pendry is a condensed matter theorist and has worked at Imperial College since 1981. He has worked extensively on electronic and structural properties of surfaces developing the theory of low energy diffraction and of electronic surface states. More recently he turned his attention to photonic materials this interest led to his present research into the remarkable electromagnetic properties of materials where the normal response to electromagnetic fields is reversed, leading to negative values for the refractive index. In collaboration with scientists at Marconi he designed a series of metamaterials, completely novel materials with properties not found in nature. These designs were subsequently the basis for new concepts with radical consequences, such as the first material with a negative refractive index, the concept of a perfect lens, and a prototype cloaking device, which have both caught the imagination of the world’s media.
Quantum Information Processing and Raising Schrödinger’s Cat
David Wineland
Tuesday, October 24, 2017
Abstract
Research on precise control of coherent quantum systems occurs in many laboratories throughout the world, for fundamental research, new measurement techniques, and more recently for the development of quantum computers. I will briefly describe experiments on these topics using trapped ions at the National Institute of Standards and Technology (NIST) but these just serve as examples of similar work being performed with many other atomic, molecular, optical (AMO) and condensed matter systems. This talk is, in part, the “story” of my involvement in these subjects which began when I entered graduate school.
Biography
David Wineland received a BA degree from the University of California, Berkeley in 1965 and a Ph.D. from Harvard University in 1970. He has been a member of the Time and Frequency Division of NIST (National Institute of Standards and Technology) in Boulder, Colorado since 1975, where he is a group leader and NIST Fellow. Starting with graduate school, a long-term goal of his work has been to increase the precision of atomic spectroscopy. This research has applications to making better atomic clocks and has led to experiments showing precise control of the energy levels and motion of atoms. Such control is now being applied to measurements whose precision is limited only by the constraints of quantum mechanics and to demonstrations of the basic building blocks of a quantum computer.
A New Type of Heat Engine, Using LEDs as Refrigerators
Eli Yablonovitch
Tuesday, October 25, 2016
Abstract
Very efficient light emitting diodes (LEDs) actually become colder as they operate because the emitted light carries away entropy. This surprising effect requires superb LED efficiency, which is achieved by using 2D photonic crystal structures to extract the luminescence. 2D photonic crystals have likewise been employed in photovoltaic cells to trap incident light. We now know that the photovoltaic cell and the LED are reciprocals of one another. The maxim that “a great solar cell has to be a great LED” has led to solar cells with record efficiency.
What if the electrical output of a photovoltaic cell drives an LED, and the LED light in turn drives the photovoltaic cell? You might fear that it would become a perpetual motion machine. Instead it becomes a heat engine in which a small amount electricity can efficiently provide refrigeration, or conversely a small temperature difference can generate electricity. Such an electro-luminescent heat engine, in which photons are the working fluid, can be more efficient than thermo-electrics, in which electrons are the working fluid.
Biography
Eli Yablonovitch is Director of the NSF Center for Energy Efficient Electronics Science (E3S), a multi-University Center headquartered at Berkeley. Yablonovitch introduced the idea that strained semiconductor
lasers could have superior performance due to reduced valence band (hole) effective mass. With almost every human interaction with the internet, optical telecommunication occurs by strained semiconductor lasers. In his photovoltaic research, Yablonovitch introduced the 4n2 (“Yablonovitch Limit”) light-trapping factor that is in worldwide use, for almost all commercial solar panels. Based on his mantra that “a great solar cell also needs to be a great LED”, his startup company Alta Devices Inc. has, since 2011, held the world record for solar cell efficiency, now 28.8% at 1 sun. He is regarded as a Father of the Photonic BandGap concept, and he coined the term "Photonic Crystal". The geometrical structure of the first experimentally realized Photonic bandgap, is sometimes called “Yablonovite”. His startup company Ethertronics Inc., has shipped over one billion cellphone antennas. He has been elected to the NAE, the NAS, and as Foreign Member, UK Royal Society. Among his honors is the Buckley Prize of the American Physical Society, and the Isaac Newton Medal of the UK Institute of Physics.
Remote Detection via Quantum Coherence
Professor Marlan O. Scully
Monday, October 19, 2015
1101 Biosciences Research Building, University of Maryland
Abstract
There is nothing so practical as a good theory. As a case in point, the compelling need for standoff detection of hazardous gases and vapor indicators of explosives has motivated the development of remotely pumped schemes that produce radiation in the backward direction.1,2 Moving from conceptualization to theoretical analysis and experimental verification, we demonstrate that high gain can be achieved in air. Backward air lasing provides possibilities for remote detection,3 as will be discussed.
Biography
Marlan Scully (Baylor, Princeton, and Texas A&M) has worked on a variety of problems in laser physics and quantum optics, including: the first quantum theory of the laser with Lamb, the laser phase transition analogy and its applications to the Bose condensate, experimental demonstrations of lasing without inversion, and ultraslow light in hot gases via quantum coherence.
His introduction of entanglement interferometry to quantum optics has shed light on the foundations of quantum mechanics, e.g., the quantum eraser. Recently he and his colleagues have applied quantum coherence to remote sensing of anthrax and probing through turbid mediums, such as skin and plant tissue.
Scully is currently a Distinguished University Professor at Texas A&M University and also holds positions at Princeton and Baylor Universities. He has been elected to the U.S. National Academy of Sciences and the Max Planck Society. He has recently been awarded the OSA Frederic Ives Medal / Quinn Prize, the DPG/OSA Herbert Walther Award, and the Commemorative Silver Medal of the Senate of the Czech Republic (by K. Chapin).
[1] Dogariu, A, Michael, JB, Scully, MO, Miles, RB, High-Gain Backward Lasing in Air, Science, 331(6016), 442-445 (2011).
[2] Svidzinsky, AA, Yuan, LQ, Scully, MO, Transient lasing without inversion, New Journal of Physics, vol. 15, art. no. 053044 (2013).
[3] Yuan, L, et al., Coherent Raman Umklappscattering, Laser Physics Letters, 8(10), 736-741 (2011).